Part accelerator, part reaction chamber, Zap’s devices are unlike any others in fusion.
Inside a Zap fusion core, a carefully orchestrated sequence of events sets the stage for fusion. Like a symphony, every step must be executed with precision, and in perfect time. This progression creates the intense conditions that lead to fusion, unlocking the potential for a transformative new energy source.
The origins of Zap’s technology can be traced back two decades to Uri Shumlak’s lab at the University of Washington. There, Shumlak theorized a new approach to fusion called a sheared-flow-stabilized Z pinch. Building on that work, he and his collaborators designed and built a series of experimental fusion devices to test his ideas.
“We started at a very low level,” Shumlak says. “But we’ve been able to show in both models and experiments that you can increase the current in a sheared-flow-stabilized Z pinch and if you do it properly, the fusion yield will also increase,” he says.
Shumlak, now Zap Energy’s Chief Science Officer, co-founded the company in 2017 alongside Benj Conway, Zap’s CEO, and Brian A. Nelson, Zap’s Chief Technology Officer. Nelson is also a UW professor emeritus who has engineered and operated every generation of sheared-flow-stabilized Z-pinch fusion device going back to the 1990s.
Today, Zap Energy is building quickly on the foundation of Shumlak and Nelson’s earlier work, with the goal of developing commercially viable fusion energy. As of 2023, the company performs R&D on two devices, named the Fusion Z-pinch Experiment (FuZE) and FuZE-Q.
Anatomy of a Z pinch
The heart of a Zap fusion system is a roughly 10-foot-long cylindrical vacuum chamber where four key steps in the SFS Z-pinch fusion sequence happen in less than one thousandth of a second, resulting in a burst of fusion energy.
Step 1: Ionize
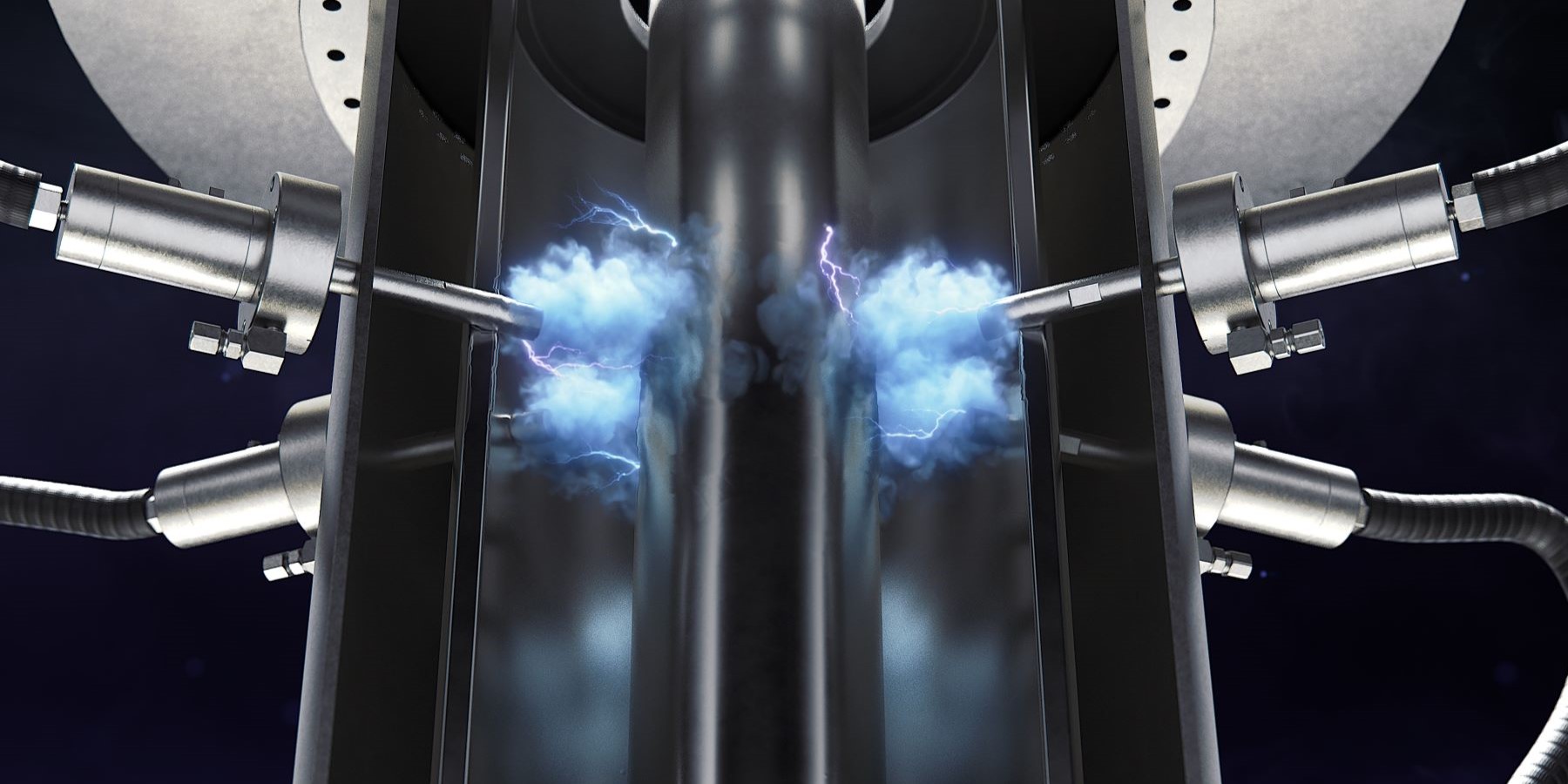
Step 2: Accelerate

Step 3: Pinch
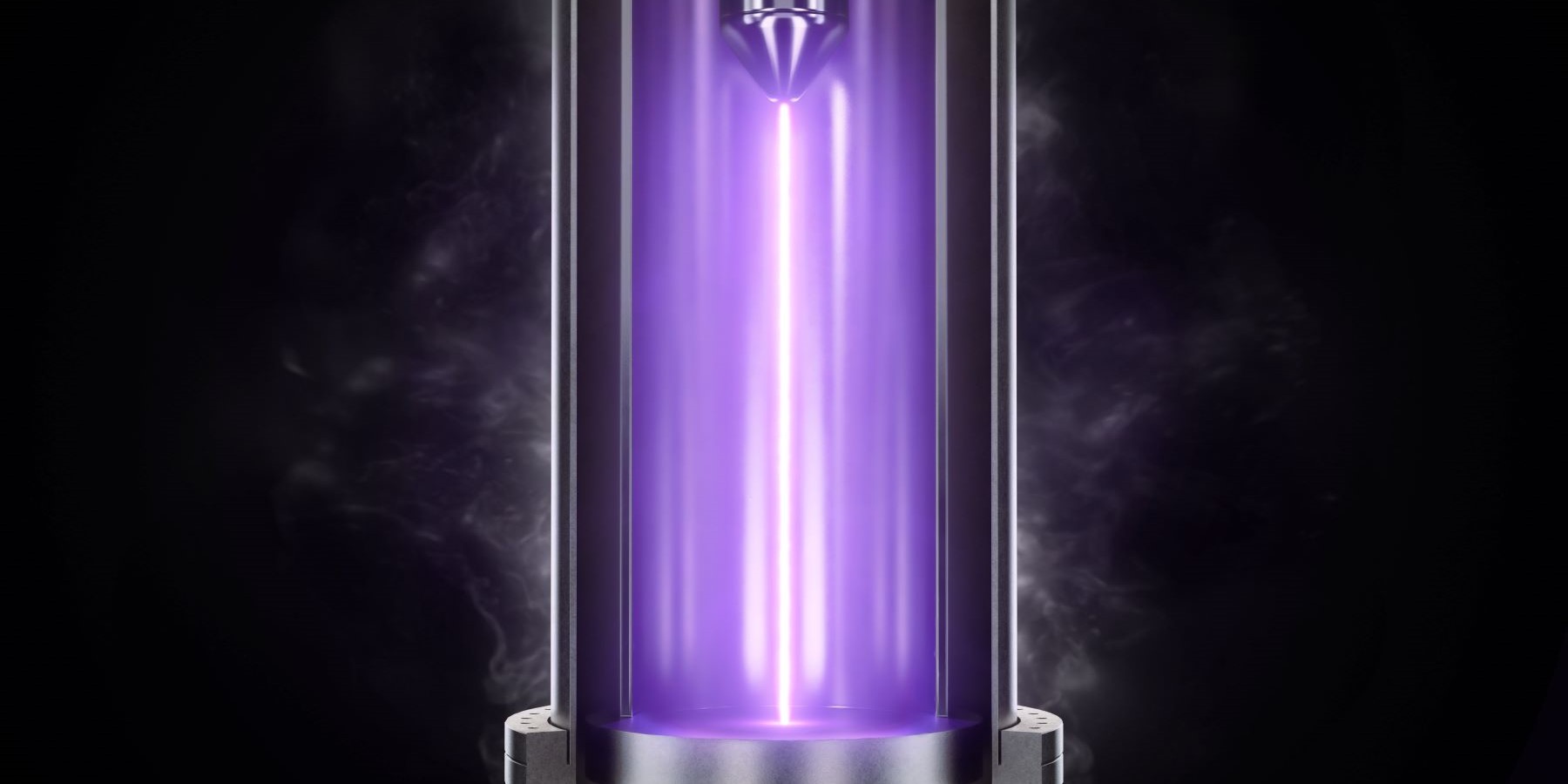
Step 4: Fuse
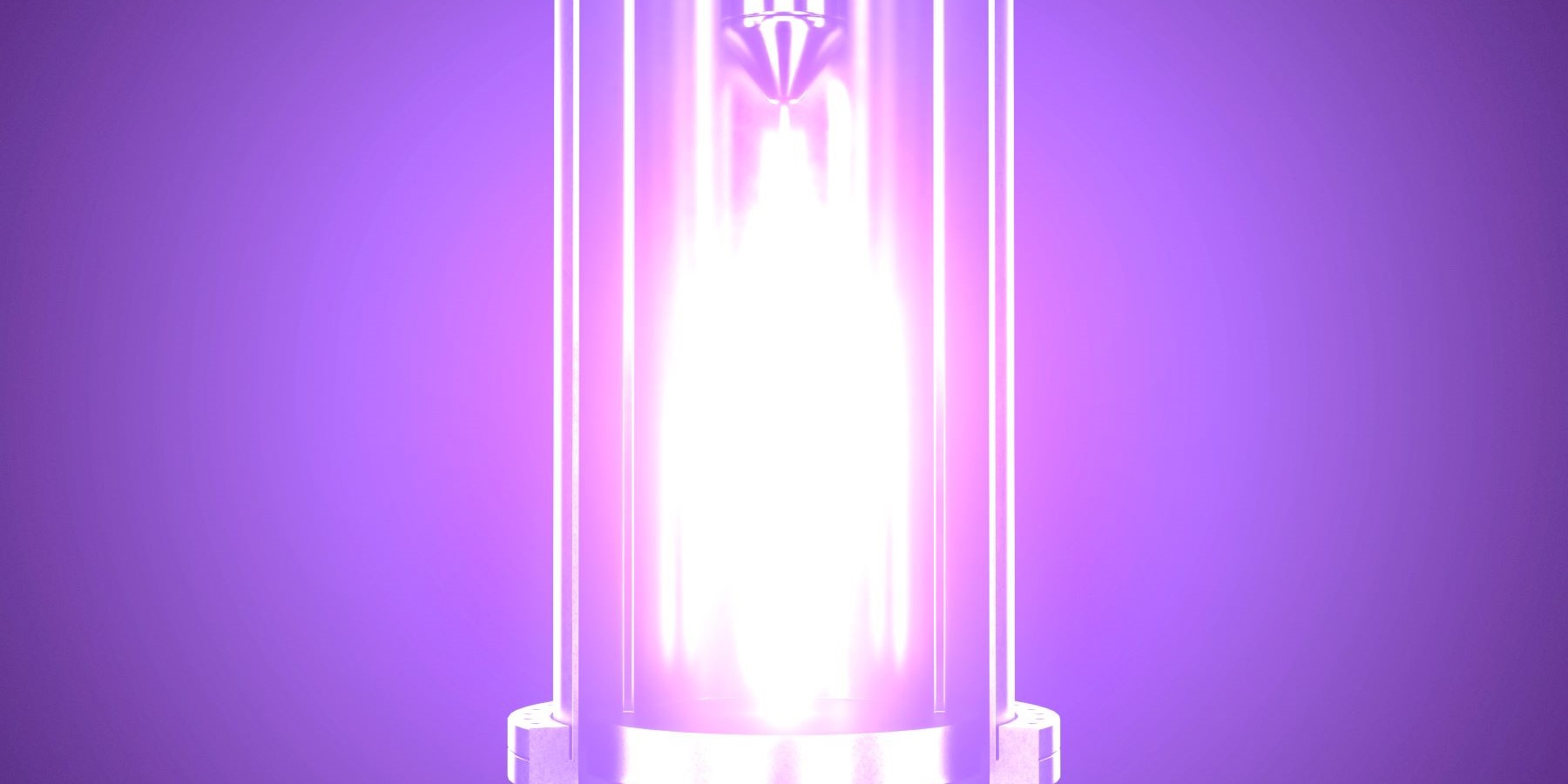
The steps are straightforward, but aligning every aspect to make sure the resulting plasma is hot and dense enough, and also stable for long enough, is extremely difficult.
“A plasma is basically this electrically charged gas that’s moving and acting like a fluid, but also heavily influenced by magnetic forces” explains Hannah Meek, a Zap Energy research engineer. “The really tricky part is that it’s incredibly unstable. Slight changes in timing, gas pressures or voltages can completely change its dynamics.”
Progress toward energy gain
Zap has already verified its approach creates fusion energy, and modeling suggests it will continue to intensify as the current in the pinch increases. The success of R&D on FuZE led to the creation of Zap’s latest-generation device, called FuZE-Q, which brings together a more powerful capacitor bank with advances in electrode design, materials, diagnostics and more. Both FuZE and FuZE-Q have already been used to create fusion plasmas thousands of times. By steadily ramping up the current in the pinch and accumulating a mountain of data along the way, Zap is moving closer to the elusive point known as scientific Q=1, where fusion power leaving the plasma equals the power put into the plasma to create the fusion conditions.
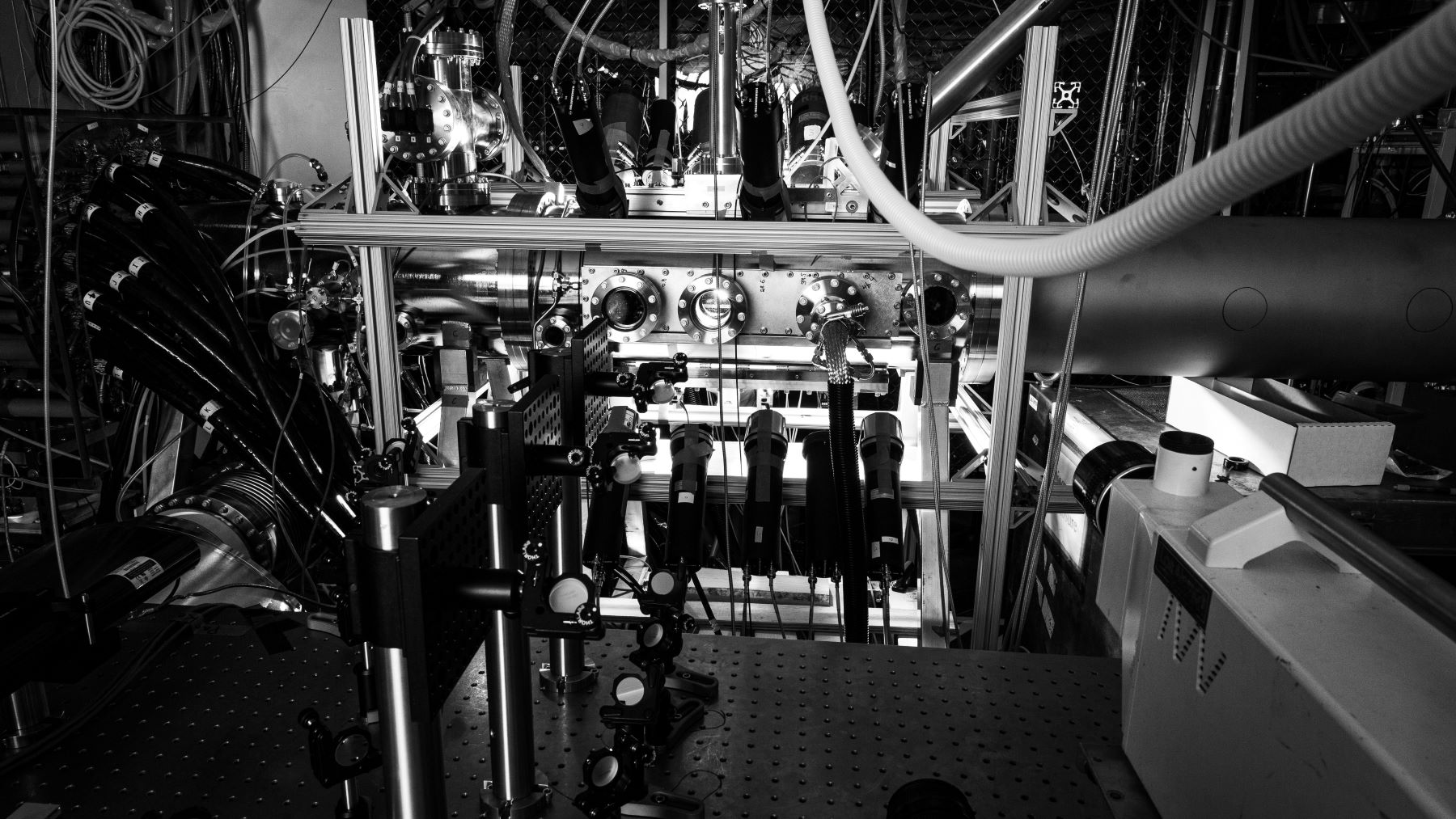
Fortunately, getting to that point and beyond benefits from a strong multiplier effect in a sheared-flow Z-pinch, says Ben Levitt, Vice President of R&D at Zap Energy. In a well-aligned and stable pinch, even a small increase in current leads to a large increase in the temperature and density of the plasma, and ultimately, how many neutrons are produced.
“That multiplier effect is exponential. Doubling the current would yield more than 2,000 times as many neutrons,” Levitt says. “In other words, we're on a really, really steep curve.”
